FNAL g-2 Experiment
In April 2021, the Fermilab Muon g-2 experiment announced a new measurement of the magnetic moment of the muon. This result confirmed an earlier result from Brookhaven Lab, and took the disagreement with the Standard Model to 4.2 standard deviations, and could be the sign of a major new discovery. Ongoing analysis will confirm this. You can see the announcement on the Fermilab YouTube channel, where you can also see another video explaining the result. Jorge Cham has also illustrated the results in an easy to follow set of cartoons.
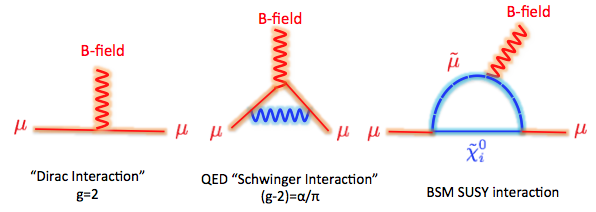
The simplest magnetic interaction of a charged particle with a B-field is described by the Dirac equation where charged particles have a magnetic moment. The magnetic moment of is defined as gsQe/2m where s is the particle's spin, m its mass and Qe its charge. In the Dirac theory, the dimensionless number g is exactly two - however interactions involving virtual particles modify this value of g by a small amount (0.1%). A precise measurement of (g-2) thus gives information about these virtual particles, and all possible particles will contribute to the shift in g. A comparison of the measured value with the current theory therefore can indicate whether there are new particles contributing to g - such as those predicted by models of physics beyond the Standard Model.
The simplest higher order interaction, the "QED Schwinger interaction", modifies g-2 by exactly α/π where α is the EM fine structure constant (1/137.04). New unknown particle contribute to the (g-2) proportionally to (m/M)2 where m is the mass of the particle and M the mass of the new particle. The measurement of the electron's g-2 is the most precisely determined quantity in physics. It has been measured to 3 parts in 1013 and its value calculated in QED from a summation of 12,672 Feynman diagrams! presently the measured and predicted values are in good agreement, indicating no new physics effects.
However, the effect of new physics scales with (m/M)2, where m is the mass particle being measured and M the mass of the new physics particles. A measurement of the muon, whose mass is 220 times that of the electron, therefore has a sensitivity to new particles with masses in the range 10 MeV to 1000 GeV (compared to < 100 MeV for the electron). The previous result for the muon g-2 used data taken in 2001 from an accelerator and muon storage ring at Brookhaven National Laboratory (BNL) on Long Island, and differed from that predicted by the SM by approximately 3.5 standard deviations. This has produced much speculation and this result is the second most cited in experimental particle physics (with over 3,000 citations).
To improve on the Brookhaven result, the FNAL experiment will determine the muon g-2 to 0.14 parts per million (the most accurate measurement of any quantity at an accelerator - for comparison, the Z mass was determined by the LEP experiments to a precision of 20 parts per million). The FNAL experiment uses the new muon beamline at Fermilab and re-uses the existing Brookhaven storage ring. This 14m diameter, 17 tonne storage ring provides a uniform magnetic field to better than 0.1 parts per million, and was transported 3000 miles from Brookhaven (Long Island) to Fermilab (Chicago) via road and barge in 2012. First results from FNAL Muon g-2 in 2021 extended the disagreement with the Standard Model to 4.2 standard deviations. Analysis of more data is ongoing to determine if this really is a harbinger of new physics.
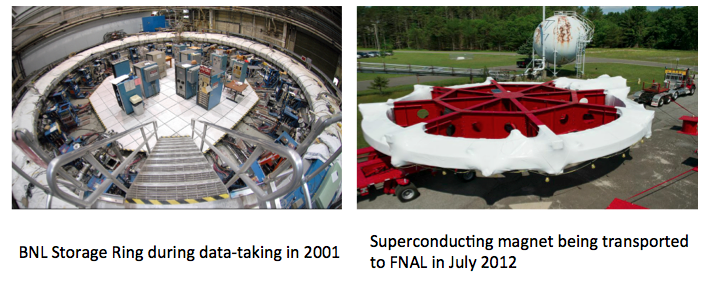
The magnetic moment is determined by injecting polarised muons of a very specific energy (3.1 GeV) into a storage ring that has a uniform 1.45 T magnetic field and counting the number of decay positrons above 1.9 GeV in 24 calorimeters around the storage ring as a function of time. The direction of the higher energy decay positrons is strongly correlated with the direction of the muon spin which precesses around the magnetic field at a rate determined by (g-2). To achieve the 0.1 parts per million in statistical precision 200 billion decay positrons must be measured by injecting more than 10,000 muons in 120ns bunches 300 million times into the storage ring over a period of 1-2 years. The muons are created from pion decays which in turn come from an 8 GeV proton beam striking a lithium target.
The UK joined the g-2 experiment in 2013, and UCL is making key contributions to the experiment in a number of areas:
- Readout, DAQ and control logic for the straw trackers
- Data quality monitoring.
- Tracking algorithm development to deal with pile-up, calibrations and increasing efficiency.
- Determination of key systematics on the muon g-2 result.
- Leading the complementary search for an electric dipole moment of the muon.
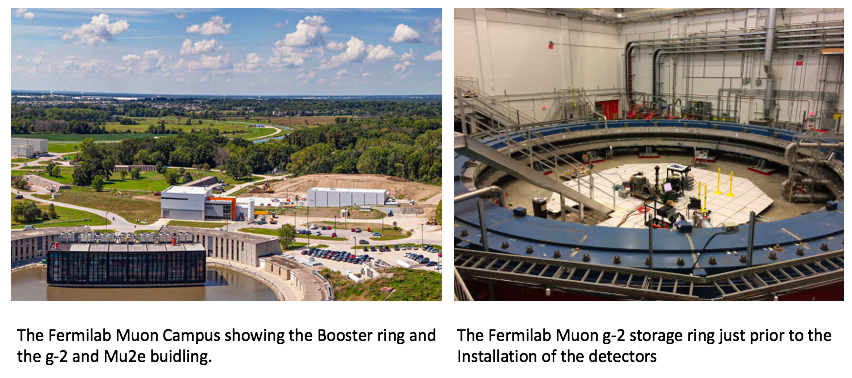