Quantum Technologies and the Absolute Neutrino Mass
Just as quantum computing promises to revolutionise the traditional computing paradigm, quantum technologies have the potential to radically change the way we do experiments in particle and fundamental physics. Quantum Technologies for Neutrino Physics, QTNM, is one of the 7 projects recently funded by the UK Research and Innovation (UKRI) under the Quantum Technologies for Fundamental Physics Programme (QTFP). Its overarching goal is to build on recent investments in quantum sensors to carry out an experiment capable of a positive measurement of the absolute neutrino mass .
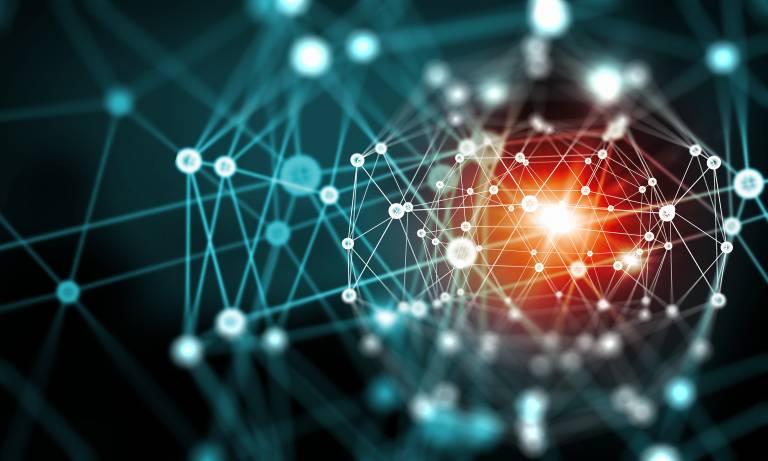
QTNM Experiment: Quantum Technologies for Neutrino Mass
Neutrinos is the most abundant matter particle in the universe. There are on average 330 of them in a cm3 . As such they play a crucial role in the evolution of the universe, the birth and death of stars and many other fundamental processes. They are however poorly understood. The discovery of neutrino oscillations provided a powerful evidence that, contrary to the initial assumptions of the Standard Model of particle physics, neutrinos have a tiny but non-zero mass.
The question of the absolute neutrino mass value turns out to be crucial for our understanding of the origin of matter and the early evolution of the universe but it remains to be the (last) unmeasured mass parameter of the Standard Model.
A laboratory measurement of the absolute mass is therefore one of the most important challenges of modern particle physics. Current leading techniques employed by the KATRIN experiment produced for the first time a sub-eV upper limit on the mass of the neutrino emitted in beta decay. This technique however cannot probe neutrino masses below 200 meV. Reaching better sensitivities is motivated by the two mass scales: one corresponding to the so called inverted mass ordering (50 meV) and the other to the normal ordering (> 9 meV). The goal of the QTNM collaboration is to use recent breakthroughs in quantum technologies to carry out an experiment capable of a guaranteed measurement of neutrino mass, even in the worst case scenario of it being ~10 meV.
CRESDA: Cyclotron Radiation Electron Spectroscopy Demonstrator Apparatus
To do this QTNM will employ a technique known as Cyclotron Radiation Emission Spectroscopy (CRES), pioneered by the Project 8 Experiment. In this approach one can determine the energy of electrons emitted in the β-decay of tritium by measuring the frequency of electromagnetic radiation generated as a a result of the electron's motion in a magnetic field. By analysing the electron spectrum near the end-point of beta decay one can infer the neutrino mass in a model independent way. Energy and momentum conservation is the only assumption!
The goal of the 3-year R&D project funded under the QTFP programme is to build a CRES Demonstrator Apparatus (CRESDA) to address the key challenges of the CRES technology. CRESDA will be operated at UCL's Quantum Measurement Laboratory with Deuterium atoms but will be "Tritium-ready". The key goals of CRESDA are as follows:
- To produce and confine D/T atoms with densities of 1012 - 1014 cm-3.
- To map the magnetic field in the detection region with a 1ppm precision using D/T atoms as quantum sensors with Rydberg atom magnetometry .
- To build a quantum limited microwave detection system in the 20-30 GHz range to enable the electron's energy measurement with a 1ppm resolution operating at or below the quantum noise level.
- To build a software and analysis framework for full atomic and CRES simulations and signal processing and to perform sensitivity calculations for a CRES-based neutrino experiment.
A schematic design of CRESDA is shown in the Figure below. The main components of the setup are: 1) the atomic source were D-atoms are produced from molecular deuterium by an electric discharge; 2) the storage ring where D-atoms will be circulated and confined; 3) the CRES magnet assembly (CMA) with a magnetic field of 1T where the cyclotron radiation from electrons is detected. In such a field a 18.6 keV electron near the end-point of the tritium beta decay will produce a microwave signal from its cyclotron motion peaked at 27 GHz; 4) the atom detection region to characterise atomic densities and velocities, and perform a 3D mapping with Rydberg magnetometry.
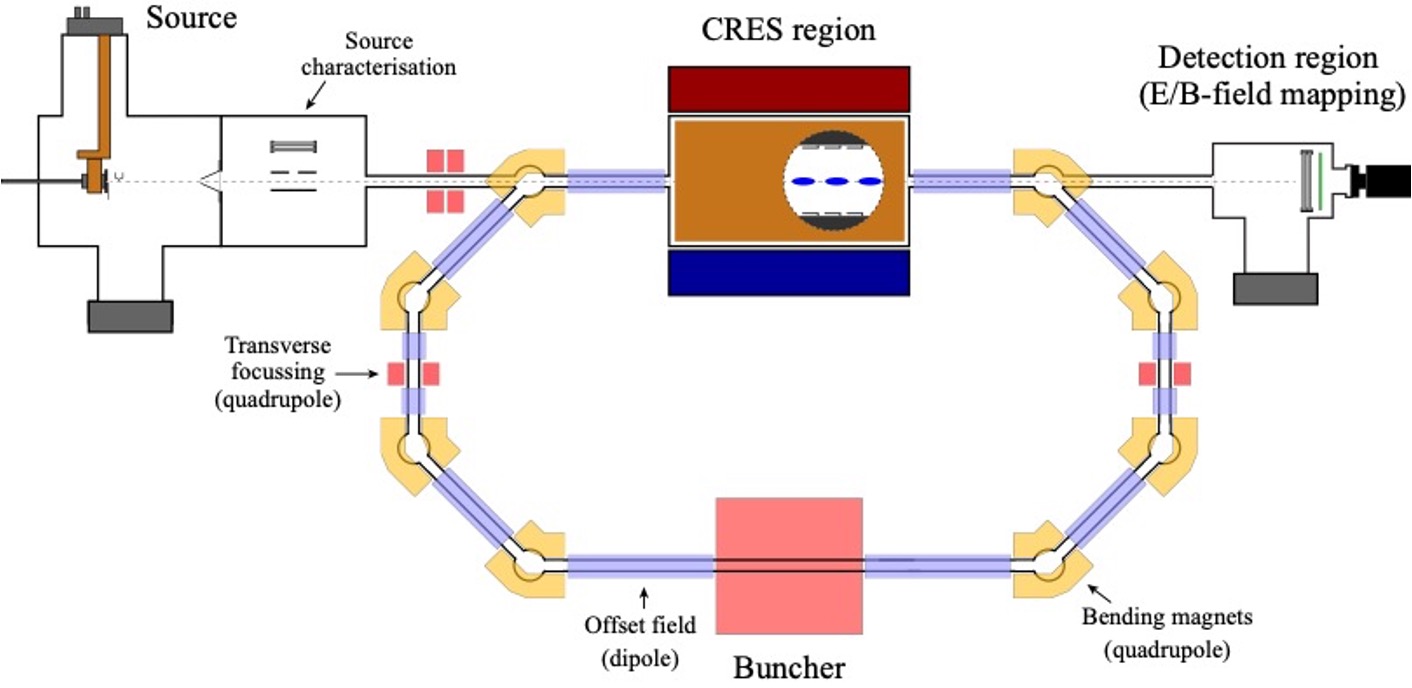
The Collaboration
QTNM is a truly interdisciplinary project that involve a close collaboration of particle, atomic and cold matter physicists, quantum sensor experts and electronics engineers. It has over 20 members from 5 UK institutions:
- UCL
- HEP Group. Contact: Ruben Saakyan (QTNM PI)
- AMOPP Group. Contact: Stephen Hogan
- University of Cambridge. Contact: Stafford Withington
- National Physical Laboratory. Contact: Ling Hao
- University of Warwick. Contact: Yorck Ramachers
- University of Swansea. Contact: Lijie Li
