A History of Early High Energy Physics Research at UCL
Jim Grozier
The Bubble Chamber Group
The bubble chamber was invented by Donald Glaser in 1952; Cyril Dodd set up the UCL group in 1955 after visiting bubble chamber labs in the USA. The group formed in parallel with the Emulsion Group, following the completion of the cloud chamber experiments on Mount Marmolada. It consisted of two faculty members, Cyril Dodd and Cyril Henderson, as well as Russell Stannard (research assistant, also working in the Emulsion Group), and Harry Tomlinson (engineer).
Cyril Henderson had done his first degree with UCL, which, because of the wartime evacuation of London, had moved temporarily to Bangor in north Wales. After release from wartime work, he took a PhD at UCL and was appointed a lecturer in 1946. He then worked on the construction of the UCL microtrons, and after three years of working on the department's beta ray spectrometer, he moved to the bubble chamber group. [1]
Fred Bullock joined the group for the remainder of his PhD, having started it on the cloud chamber work. In 1956 George Kalmus - brother of Peter Kalmus - started a PhD under Dodd and Henderson on The Physical Properties of Bubble Chambers. [2]
Dodd's first bubble chamber used pentane, as had Glaser's. But whereas Glaser had managed to see tracks, Dodd's chamber simply filled with bubbles. Eventually it was realised that British pentane, being derived from wood alcohol, contained too much 14C to be used in a bubble chamber. US pentane came from petroleum, in which the 14C had had time to decay to an acceptable level. (It is rumoured that Glaser first tested his idea by shaking a bottle of beer, then holding it near a radioactive source to see if the number of bubbles would increase!)
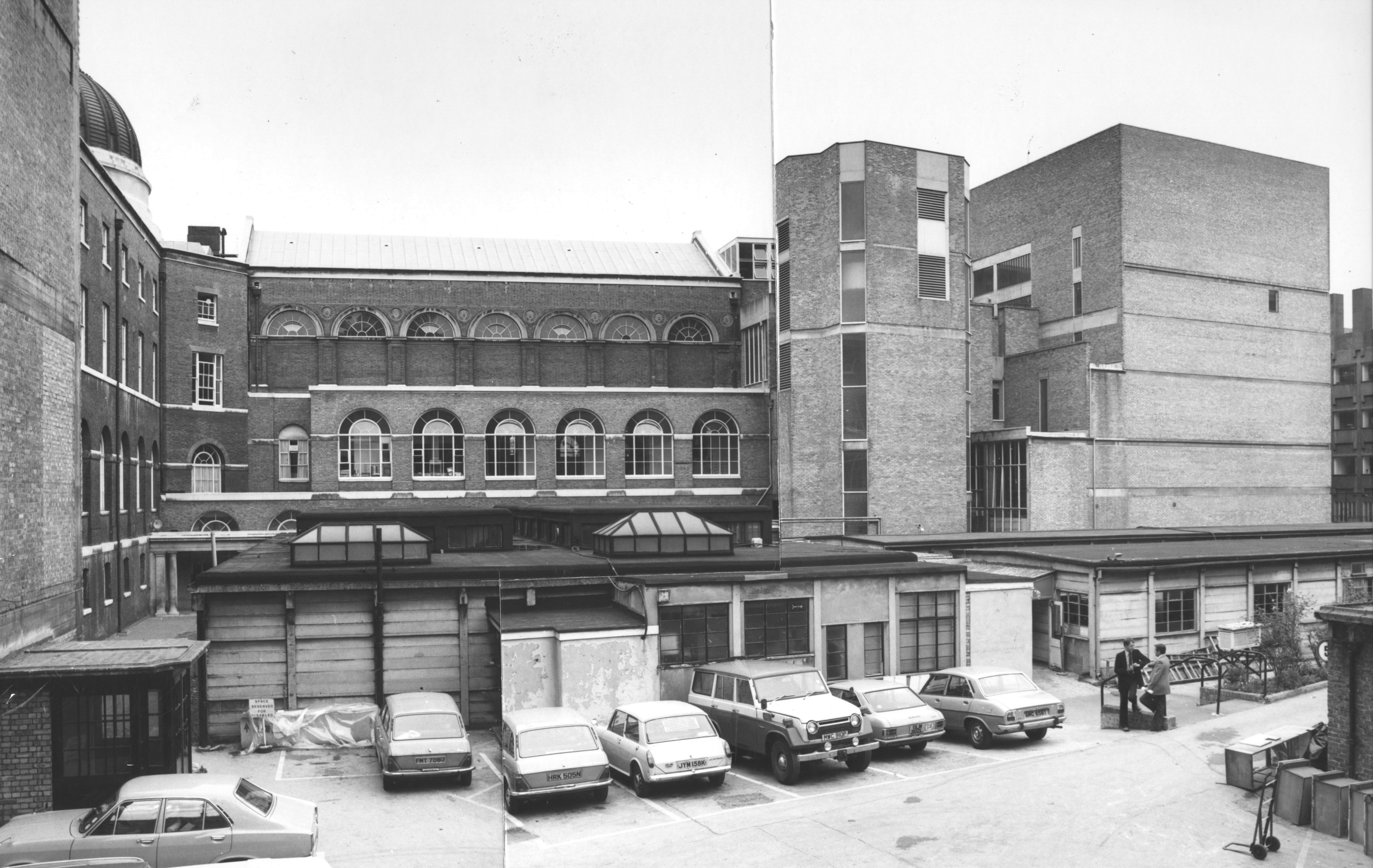
Fred Bullock saw Dodd as "an old-time physicist" who did not really understand the new techniques, although other contemporaries, such as Cyril Henderson, disagree. At any rate, Bullock felt that he was working on his own and that Dodd had lost interest, although George Kalmus points out that Dodd was by then Senior Tutor, and thus was very busy. Bullock and Kalmus built a small bubble chamber and installed it in one of the temporary post-war huts in the area behind the South Cloisters, where the Biochemical Engineering building now stands. The bubble chamber worked, but caused what Bullock describes as "some disquiet" in neighbouring parts of the College. This was because the chamber had to undergo a sudden expansion in order to produce tracks, and the expansion "produced an exceptionally loud explosion". Following complaints about the noise, the lab was visited by the Provost and the Bursar; luckily the physicists managed to convince them that this was the forefront of particle detection technology - and they were allowed to continue. [2]
A Dangerous Experiment
At that time it was thought that the future of bubble chambers lay in the use of very dense liquids. So the UCL group set about building one using the densest known liquid with a boiling point in the right range for a bubble chamber - tungsten hexafluoride. This was certainly a challenging project - as well as being intensely poisonous, the gas disintegrates into hydrofluoric acid in the presense of water. Hydrofluoric acid attacks glass, so the entire chamber (apart from the viewing window, safely under the liquid surface) had to be made from monel metal, an alloy of copper, nickel and other metals which is resistant to HF.
Fred Bullock, who was involved in this experiment along with George Kalmus, recalls that this challenge was welcomed by the UCL workshop, who had to create new methods of welding and bending the unfamiliar material. The apparatus was built, and installed in one of the huts. All went well, until one day one of the pipe joints sprung a leak. White clouds of hydrofluoric acid were seen escaping. The lab was evacuated, and all the physicists could do was watch through a window which gradually crazed over as the acid attacked it. Needless to say, tungsten hexafluoride was not used again! [2]
Following the completion of his PhD, Kalmus worked as a postdoc at UCL, designing the electromagnet for a heavy liquid bubble chamber to be installed at the National Institute for Research in Nuclear Science (NIRNS) - later renamed the Rutherford Laboratory. By now the material of choice for these was propane; such relatively dense liquids were chosen in order to enhance the detection of gamma rays by conversion to electron-positron pairs. Several of the UCL team spent time at Berkeley, learning about bubble chambers from Wilson Powell, including Henderson in 1958, Stannard in 1959 and Kalmus in 1962.
Henderson became the leader of the bubble chamber group, and from 1958 began to build up both its material and human resources. Kalmus returned to UCL only briefly, then went back to Berkeley for several years. One reason he cited for this move was that at that time UCL seemed to him to be "in the doldrums" whilst Berkeley was "absolutely jumping" with new ideas and discoveries. After Berkeley, he says, UCL was like going back in time; symptomatic of this lethargy was the refusal of the high energy physicists' request for their own computer - a professor of theoretical physics apparently responded by asking "What do you want one of those for?" [3]
World War Two and the "Brain Drain"
Cyril Henderson has pointed out that any comparison between UCL and Berkeley at that time needs to take account of the fact that in Europe "there had been a devastating war, taking scientists away from their labs. UCL physics labs were destroyed. Meantime there was no such interruption in the USA". He recalls from his own time at Berkeley that "there was an air of extraordinary excitement above that of any other physics department in the world". [1]
CERN and Harwell
The late 1950s saw the start of a collaboration with the Atomic Energy Research Establishment at Harwell to send a team to CERN to work on two experiments involving the first accelerator there, the 600 MeV synchrocyclotron. A grant was obtained which enabled the recruitment of technical staff and a research assistant, to be based at CERN.
The UCL NIRNS/Rutherford Lab Heavy Liquid Bubble Chamber
Note: Since this is a history of High Energy Physics at UCL, the various projects undertaken, sometimes contemporaneously, are distinguished by the sites where they were located, which were often not at UCL. Hence, for example, the bubble chamber that was built by UCL but installed at NIRNS is referred to as "the NIRNS chamber" to distinguish it from other bubble chambers built by UCL for other locations. However, as David Miller has pointed out, this chamber was very much a UCL project and was known as "the UCL chamber" by NIRNS, to distinguish it from other chambers at NIRNS built by other institutions.
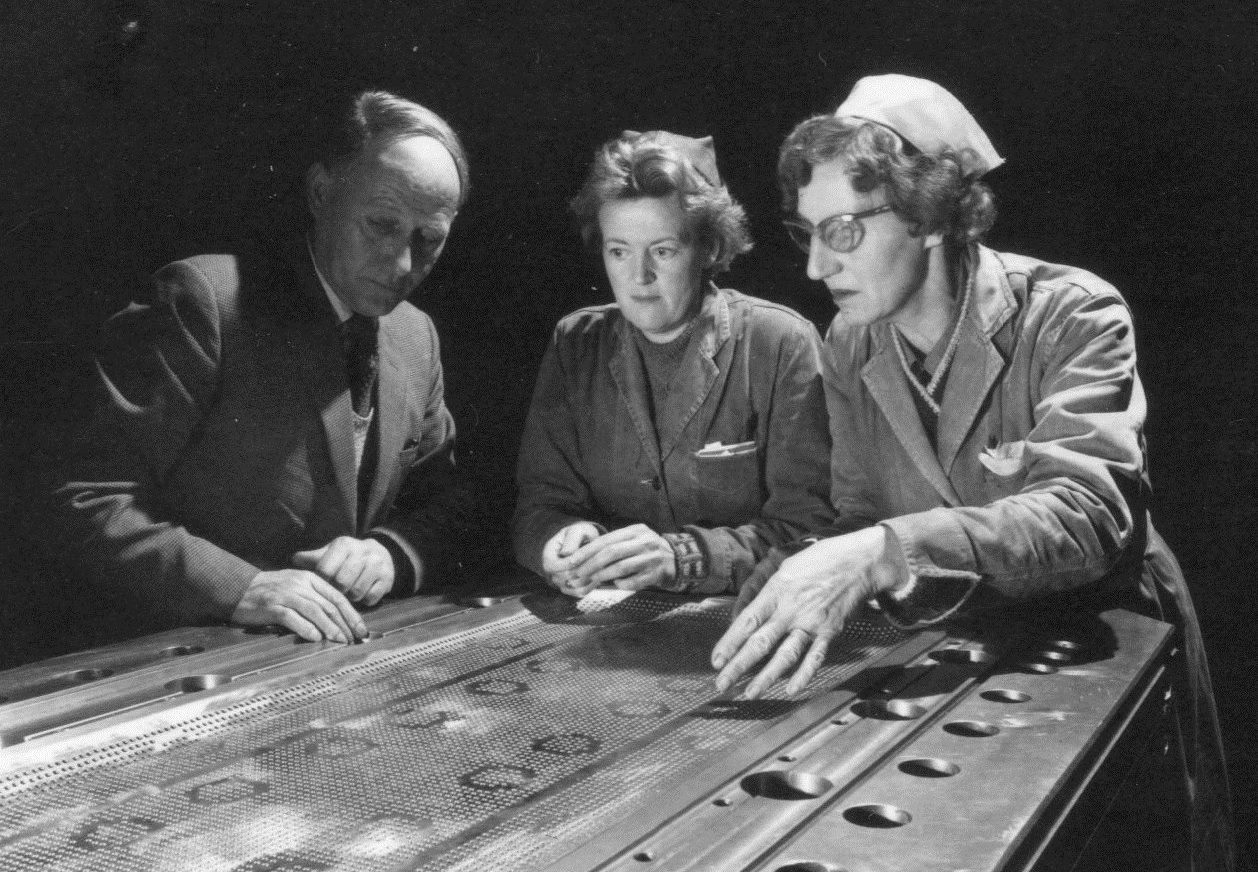
The chamber was one of three commissioned by NIRNS to work with the new 2-3 GeV NIMROD proton beam, the other two containing liquid hydrogen and liquid helium respectively. The machine was designed by a small team at UCL consisting of Harry Tomlinson, Bill Towlson, Tom Venis and three draughtsmen. The constituent parts were then made by various companies, in the UK and elsewhere; it took 5 years to build and was completed in 1965.
Bill Towlson returned to UCL in 1960 to work on this project; he had done his BSc and PhD at UCL between 1951 and 1957, in the same year as Bullock and Peter Kalmus, then worked "outside" for a few years.
The chamber was cuboidal in shape: 1.4 metres long, 50 cm high and 50 cm wide. It was kept pressurised by compressed gas, separated from the chamber itself by a movable rubber sheet with an adjacent porous metal backplate on the "gas" side. The compressed gas pushed the sheet into the chamber; the gas pressure was then quickly released, so that the sheet was forced against the backplate, expanding the liquid in the bubble chamber. The chamber was surrounded by a huge water-cooled electromagnet which weighed about 200 tons and consumed about 4MW of power. [1, 4]
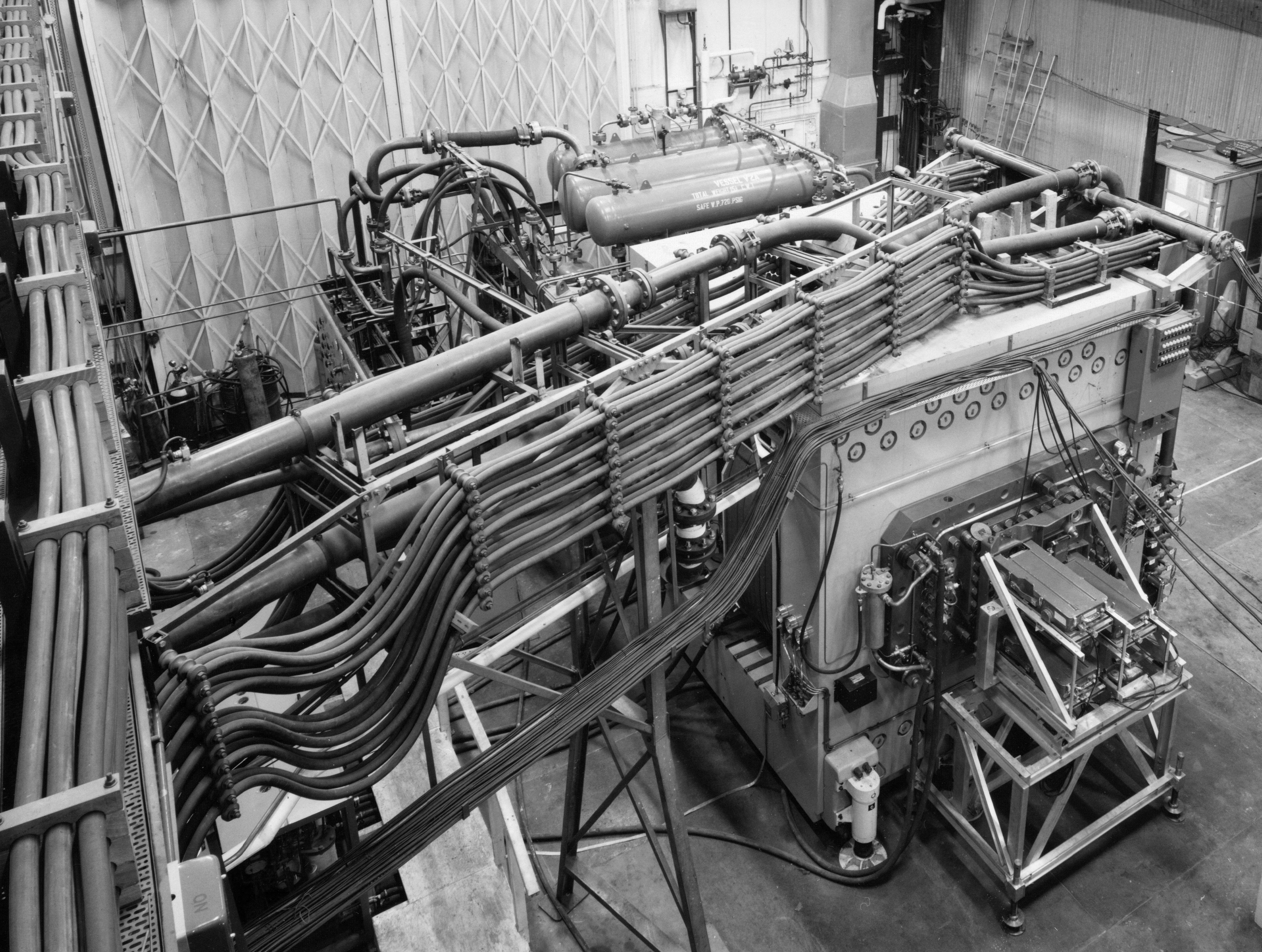
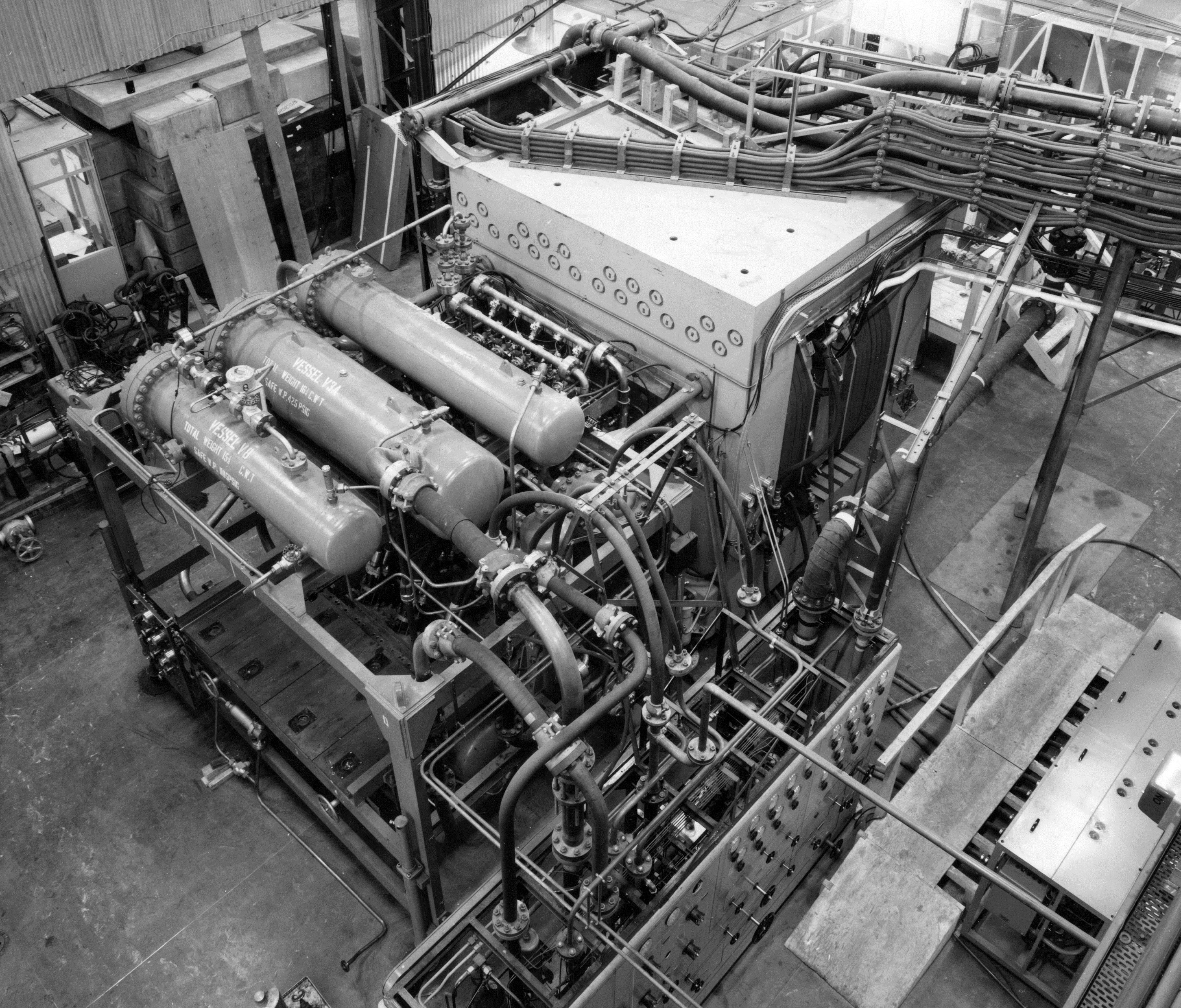
The bubble chamber was required to be movable around the experimental floor - in order to align it with various beamlines, then move it out of the way when another experiment needed the beam - and Kalmus and others achieved this using an "air pad" system similar to one that had been pioneered at CERN. The system used technology then being developed on the Isle of Wight for the first hovercraft. [4]
The completed bubble chamber was put through its paces by the design team in a commissioning run which produced 200,000 pictures, then handed over to the NIRNS staff. Brian Luetchford recalls one incident that had clearly not been anticipated:
"The chamber with its magnet stood in the middle of a huge hall which had metal lockers around the walls. When the magnet was switched on, all these lockers decided to go in towards the middle - there was so much stray field! It was well designed, but there was still a lot of stray field. You had to be careful if you were walking around near the chamber with a piece of kit, if it was made of iron ..." [5]
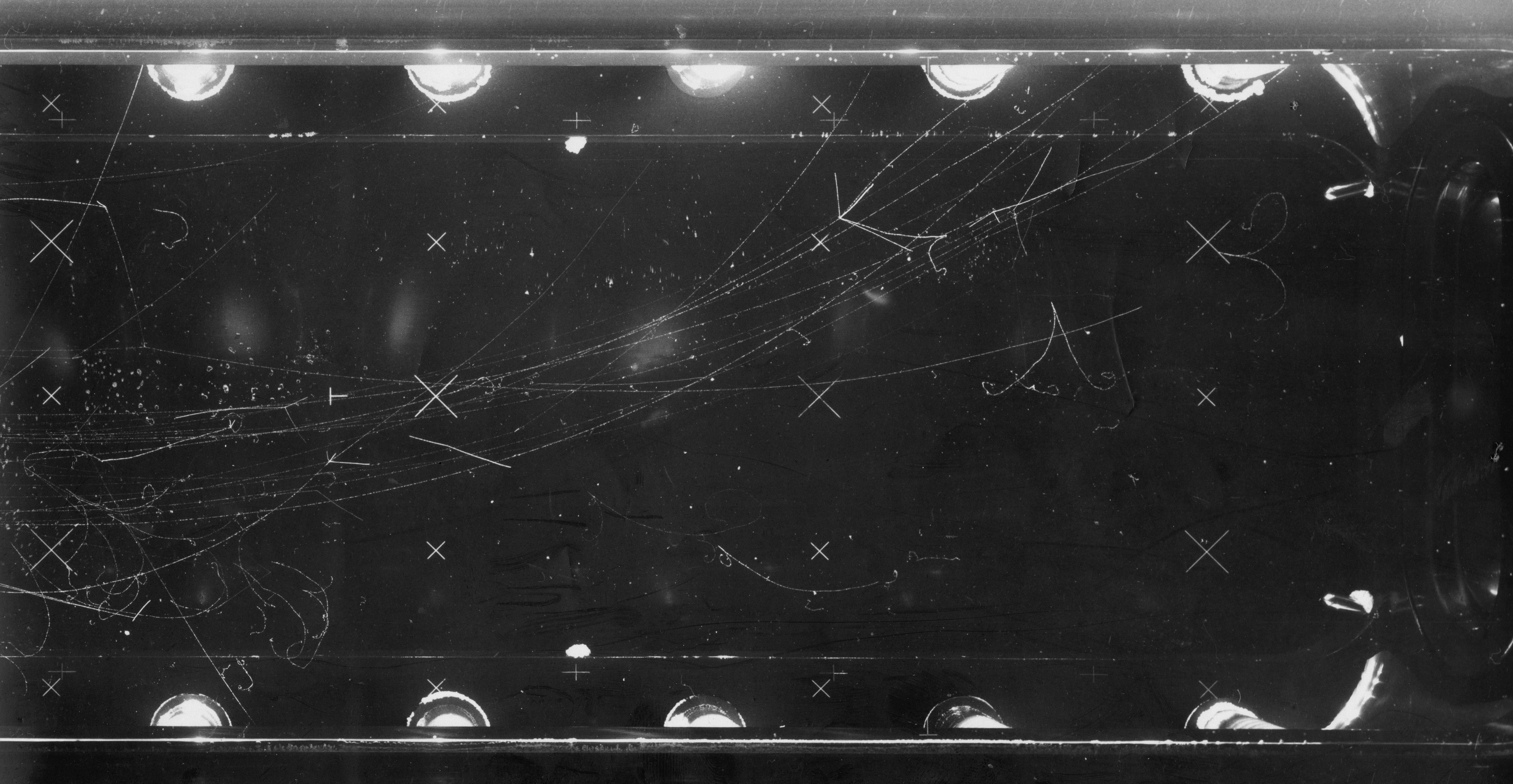
Click here for more pictures of the HLBC.
By the mid-1960s the Bubble Chamber Group consisted of Henderson plus three other members of the academic staff (Russell Stannard, Fred Bullock and Mike Esten), three postdoctoral fellows (including Bill Knight and David Miller), six PhD students, an electronics engineer (Fred Damodaran), a computer expert (Gerry Cooklin) and six scanners. Together with the Emulsion Group, the Bubble Chamber Group was "overseen" by Eric Burhop from the mid-1960s, and in 1966-67 these two merged into a single group with three sections - responsible, respectively, for emulsion work, the NIRNS bubble chamber, and Gargamelle. In October 1964, Massey wrote to I.A.Learmouth at the Department of Scientific and Industrial Research in support of a grant application submitted by Cyril Henderson for an expanded bubble chamber group. In the letter, he describes the UCL group as "the major one in this country concerned with experiments using heavy liquid chambers".
Henderson left UCL for Aberdeen University in 1967, accompanied by Fred Damodaran. Russell Stannard left two years later to help set up the physics department at the newly-constituted Open University. By this time, discussions were ongoing at CERN on plans for what became known as "Europe's last two giant bubble chambers" - Gargamelle and BEBC.
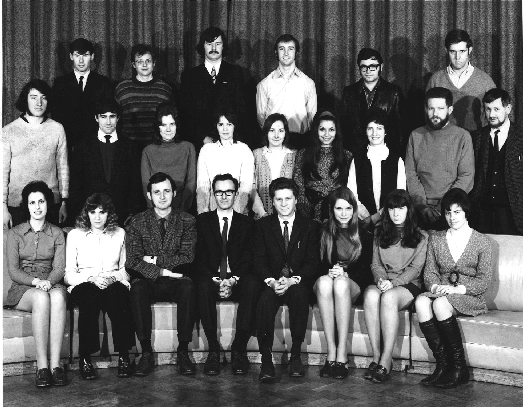
The Gargamelle Experiment
Gargamelle is widely regarded as the UCL group's finest hour. The group played a leading role in a small (by modern standards) collaboration which made a discovery that, it is said, only missed winning a Nobel prize because of the untimely death of the collaboration's acknowledged leader, André Lagarrigue. The experiment is described in some detail by Peter Galison in a 1983 paper [11 pp 477-482]; it is well-known not only within the particle physics community, but, thanks to a landmark 1988 paper by Bogen and Woodward [6], in the philosophy of science community too.
This very incomplete account of the Gargamelle experiment has been written with assistance from Mike Esten, a member of the Gargamelle collaboration.
The Chamber
In the early 1960s, particle physicists in Europe were anxious to compete with the USA, which had achieved most of the major discoveries in the field at that time, and was planning the construction of a huge heavy liquid chamber. The CERN community was divided; some members wanted to build a heavy liquid chamber, others preferred liquid hydrogen. The two fillings each had their advantages and disadvantages. The low nuclear density of the hydrogen chamber offered more precise measurement, whilst the heavy liquid chamber offered much better gamma ray detection (i.e. π0s) and – crucially for neutrino studies – it offered a greater number of interaction lengths within a chamber of a given size (i.e. far better muon detection) plus a much greater target mass (i.e. more neutrino events). In short, for a chamber of any feasible size, a heavy liquid chamber was the only choice for almost all neutrino studies.
Both heavy liquid and hydrogen chambers were eventually built; but Gargamelle, the heavy liquid chamber, was ready first, being less complicated and expensive than the hydrogen project, and furthermore, the French, in the form of the CEA (Commisariat a l'Energie Atomique) and the Ecole Polytechnique, were prepared to pay for and build it. Gargamelle was installed at CERN and under test by the end of 1970 [7]. The chamber was cylindrical in shape, 4.8 metres long and 1.9 metres in diameter, and contained 18 tons (12 m3) of freon, CF3Br. It had 8 cameras arranged in pairs, with each pair giving a stereoscopic view of a section of the cylinder, and a 2 tesla magnetic field.
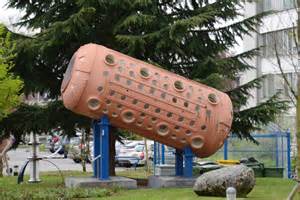
The Collaboration and the UCL Team
UCL joined six other institutions - Aachen, Brussels, CERN, Ecole Polytechnique, Milan and Orsay - in the Gargamelle collaboration in 1967. The UCL Gargamelle group was a sub-group of the Bubble Chamber Group and included Mike Esten, Fred Bullock, Tegid Jones, John Mackenzie and John Bartley [8]
The Experiment
Gargamelle was originally proposed mainly to search for the charged intermediate vector boson (the W) by studying neutrino/nucleon collisions. The events to be studied were the so-called “charged current” (CC) events in which a muon neutrino turned into a muon, and which would provide data on cross-sections and structure functions. Up until the late 1960s it was firmly believed by almost everyone that weak neutral currents simply either did not exist, or only occurred at an extremely low rate. The experimental lower limits on neutral currents in decay processes had been forced down to very low rates indeed, and the limits in scattering processes had not really received much attention, partly due to experimental difficulties and partly due to the fact that theoreticians felt no pressing need for neutral currents anywhere.
By 1970 Gargamelle construction was nearing completion, and several other objectives became included in the experimental proposal. Firstly, following recent results from SLAC which suggested a “parton” model for the nucleon, it was decided to add an investigation of proton structure via quasi-elastic and deep inelastic muon neutrino and anti-neutrino scattering events. Additionally, but very low on the list of priorities, a search was proposed for “weak neutral currents” (NC) in which a neutrino scattered off an electron in the target (a leptonic neutral current) or from a hadron (a hadronic or semi-leptonic neutral current). However, by late 1971, priorities were changed by t’Hooft’s demonstration that the SU(2) model of Weinberg and Salam (1967), which predicted the presence of neutral currents, was renormalisable and hence acceptable. Hitherto the Weinberg-Salam model had been just one among many proposed theories – all of them non-renormalisable, and to which little attention had been paid. Now it was decided to look for weak neutral currents [9].
The problem was whether it was feasible to disentangle semi-leptonic NC events from the high background rate of events caused by neutrons in the neutrino beam. These neutrons arose from beam neutrino interactions in the shielding, chamber magnet etc causing events with no muon, i.e. background NC events. One could also search for leptonic neutral current events for which the background was very low, but unfortunately the rate was also expected to be extremely low.
Protons from the 19 GeV Proton Synchrotron were directed at a target which produced pions and kaons, decaying to muons and muon neutrinos at a peak energy of about 2 GeV. A neutrino horn carrying a reversible electric current allowed either neutrinos or antineutrinos to be selected. The main signature event, on the hypothesis that semi-leptonic neutral currents exist, would be a hadron shower caused by the interaction of an incoming neutrino with a nucleon in the freon. These could be distinguished from charged-current events because the latter would also emit a muon.
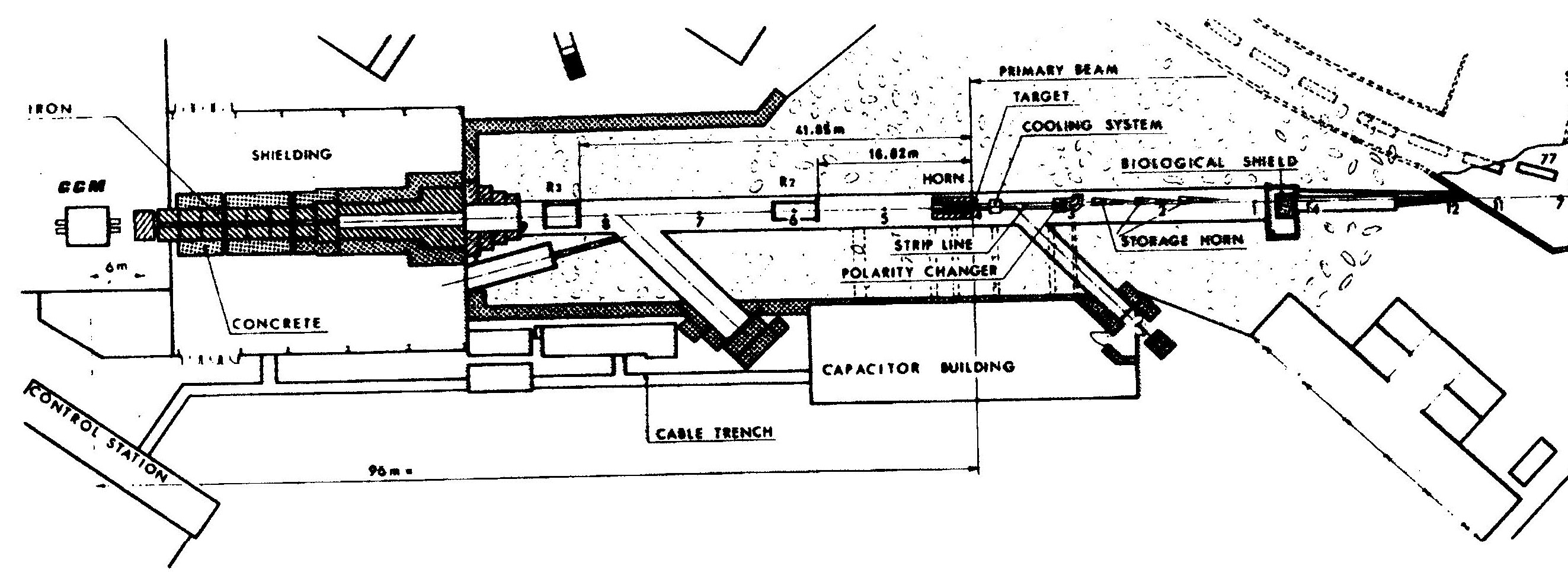
However, under the alternative hypothesis that there are no neutral currents, one would still expect to see NC-like events, where a charged-current interaction has taken place in the container walls or the shielding, producing a neutral particle which then interacts with a nucleon inside the detector, the muon having escaped undetected. It is also possible for both these interactions to occur in the freon, in which case one would see two events, one with a CC signature and one apparent NC event; for this reason, such events are called associated events (AS). The Gargamelle team's task was to show that more NC-like events are seen than can be accounted for on the hypothesis that there are no true neutral currents. [10] (There were also the rare single-electron events - leptonic neutral currents - where a neutrino interacts with an electron which then produces a track; but there would not be enough of these to compile useful statistics).
There was competition, in the form of the HPWF (Harvard-Pennsylvania-Wisconsin-Fermilab) collaboration in the USA, who were also looking for neutral currents. Gargamelle produced 83,000 pictures, all of which had to be scanned for candidate events. The pictures were shared among the collaborationg institutions, all of which had scanning teams using purpose-built machines. The UCL scanners were mainly young people such as school leavers or students in their "gap year" who had been taken on specifically to work on scanning Gargamelle film, and were trained on the job. UCL had three of these machines, manufactured by Saab, two of which were installed in the area now housing the HEP Group's meeting room, library and kitchenette (D17) and one in the room next door (D15), now an office. Scanning activities were intensified after the Aachen team found a single-electron event in January 1973; at one time there were 30 scanners at UCL, and measuring activities sometimes even continued through the night.
Scanning
The 70mm film from the bubble chamber was inserted into the machine, which projected the photographs onto a glass horizontal table, at which the scanner worked. S/he worked methodically through the eight pictures of each event, examining first the two stereoscopic views of one end of the chamber, then moving on to the next, and so on. Details of any events seen would be put into a scan sheet. This process would then be repeated by a different scanner. The results of these two scans were then transferred to punched cards for inputting to a computer, and arbitrated by a physicist.
Any interesting events, whose vertices were inside the 'fiducial volume' (i.e. not too near the chamber walls), would then be traced onto paper, and measured. The coordinates of the various vertices and tracks were recorded electronically by means of a 'puck' which was like an early mouse - it moved around the table, followed underneath by a magnet attached to wires wound on wheels which thus rotated as the puck moved. These co-ordinates would then be fed into the computer, which worked out what types of particles were involved and produced a large print-out. The measurements would again be checked by a physicist, and if necessary, repeated.
The Result
In June 1973, it was rumoured that HPWF had seen an NC signal; by this time, Gargamelle had accumulated a reasonable amount of data, and it was decided to publish this and the single-electron event; so two papers were produced [9,10]. However, in November, having modified its apparatus and started a second run, HPWF withdrew the claim, since it now appeared that some CC events had been wrongly classified as NC due to insufficient muon acceptance. (Because of the on-off nature of HPWF's claim, it was referred to within the Gargamelle community as the "alternating neutral current"!)
The Gargamelle paper showed clear evidence of neutral currents, but HPWF's 'on-off' debacle had muddied the waters to such an extent that it was some time before all physicists were convinced.
In 2009 the Gargamelle Collaboration was awarded the European Physical Society's High Energy and Particle Physics Prize for the observation of weak neutral currents. Some members of the team appear in the group photograph above.
More on Gargamelle can be found in the CERN Courier archives, including an article by Don Perkins and one by Donald Cundy and Christine Sutton.
For further details of the Bubble Chamber Group see extracts from Bill Fox's History.
References:
- Cyril Henderson, in correspondence with the author, January and April 2015
- Interview with Fred Bullock, 15 November 2013
- Interview with George Kalmus, 11 February 2013
- Interview with Bill and Pamela Towlson, 15 January 2014
- Interviews with Brian and Pat Luetchford, 9 July and 24 September 2014
- Bogen & Woodward, Saving the Phenomena. Phil.Rev. 97 No. 3 (1988) 303-352
- Dominique Pestre, History of CERN (vol. III) chapter 2
- Interview with Janet Fraser, 26 November 2012, and ongoing correspondence
- Tegid Jones, UCL HEP seminar, 21 January 2014
- Nuclear Physics B 73 (1974) 1-22
- Peter Galison, How the First Neutral-Current Experiments Ended. Rev Mod Phys 55 (2) 1983, pp 477-509