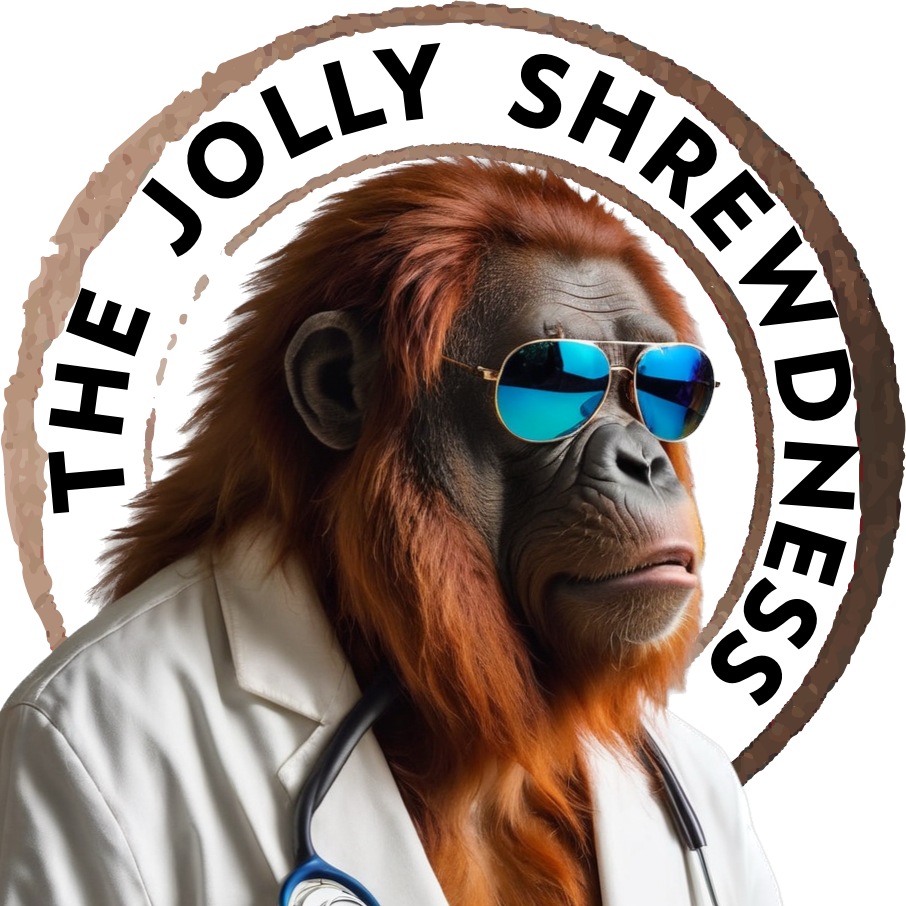
PBT Research Projects
Current Research
QuARC: Quality Assurance Range Calorimeter
Comprehensive Quality Assurance (QA) is necessary at every clinical PBT centre: verification of the Water Equivalent Path Length (WEPL) that defines the proton range in tissue, is required daily, in the hours immediately preceding the start of treatment, to ensure patients are treated safely. Such measurements need to be highly accurate — to better than 1 mm — but also reproducible to set up and efficient as pressure exists to maximise time available to treat patients. Existing QA systems are frequently time consuming to set up and either expensive and fragile or only able to perform basic range verification, ie. for only a few predefined proton energies. The ideal detector would allow measurements of the necessary resolution to be carried out in a matter of minutes with minimal setup time to reduce time constraints on clinical staff.
The development of a detector — the Quality Assurance Range Calorimeter (QuARC) — is underway at UCL to develop a clinically-ready prototype detector for making fast, sub-millimetre WEPL measurements. This forms part of an R&D programme that builds on over a decade of experience on the SuperNEMO neutrinoless double beta decay experiment. A stack of plastic scintillators — originally developed for the SuperNEMO calorimeter — intercepts the beam: the dose deposition with depth is obtained directly from the resulting scintillation light from each sheet. Since the density of plastic is extremely close to water, the WEPL can be measured directly. By reducing the setup and measurement time and improving the accuracy when compared to the existing market-leading solutions, more time can be made available for patient treatment at each clinical PBT centre, thereby increasing access for patients.
QuADProBe: Quality Assurance Detector for Proton Beam Therapy
Building on the development of the QuARC, a more sophisticated detector — the Quality Assurance Detector for Proton Beam Therapy (QuADProBe) — is also under development to make fast, accurate measurements of proton beam spot position, size and range in water for both daily machine QA and patient-specific treatment plan verification (PSQA).
Past Research
Designing Convolutional Neural Networks for Scintillation Photography
Modern radiotherapy treatments provide complex dose distributions which are difficult to measure and verify. This is due to beams having high-dose gradients, time-varying intensity and sizes reducing to millimetre scales. A dosimeter was designed to provide improvements over standard detectors that are unsuitable for measuring complex small fields. The proposed detector system consists of an irradiated scintillator sheet that is photographed, from which the dose is reconstructed. This provides a cheap, fast, and high-resolution solution. But scintillation images come with a variety of visual artefacts that need correcting.
Convolutional neural networks (CNN) have been shown to have excellent accuracy in extracting useful information from noisy images. This requires thousands to millions of training images. In scintillation photography, there is not enough data to achieve an acceptable performance for CNNs. A novel method using domain randomisation was used to solve this issue, where thousands of images were simulated with varying physical parameters. This data can be used to train the CNN to be robust to visual artefacts. These CNNs are designed to assist in our image processing by predicting relative dose distributions and (un)known physical parameters, which gives confidence that the measured images are correct. Results showed that CNNs performed better than classical methods and could provide dose distributions that are suitable for routine QA.
Iterative Reconstruction Using Structural Priors in Proton Computed Tomography
Proton computed tomography is an imaging technique showing high potential in the reduction of errors in proton therapy treatment planning as it probes the relevant quantity — the relative stopping power — directly and avoids introducing errors via additional calibration steps. Protons do, however, undergo multiple Coulomb scattering as they travel through materials, leading to inherently blurry images. The quality of the image reconstruction in proton CT imaging can be improved with the use of structural priors, where x-ray CT images provide a reference guiding the reconstruction in the form of a regularising term in the objective function of the inverse problem. A structural prior known as the directional total variation can be compared to a baseline provided by a “standard” total variation regularisation in reconstructions obtained using a FISTA-type algorithm. This provides a clear advantage in terms of spatial resolution.